Towards hybridizing and intensifying electrochemical disinfection techniques
DOI:
https://doi.org/10.57056/ajet.v9i1.160Keywords:
Disinfection by-products, Antibiotic-resistant bacteria, Electrocoagulation (EC), Electrolytic water splitting, Antibiotic-resistant genes, Reactive oxygen speciesAbstract
Halting the transmission of waterborne diseases relies heavily on disinfection, a crucial barrier against pathogenic microorganisms. Electrochemical disinfection (ED) has emerged as a widely studied and implemented approach for effectively neutralizing these harmful microorganisms in water and wastewater. This is primarily due to ED's simplicity, efficiency, and environmentally friendly nature. This review provides a concise overview of ED's fundamental mechanisms and systematically examines the targeted species. Furthermore, we comprehensively explore the explore the actual employment of ED in dealing with water and wastewater. Lastly, we discuss the potential for combining ED with other technologies and synergies, laying the groundwork for future engineering advancements. The literature primarily focuses on various ED methods for eliminating pathogens. Still, there is limited understanding of how process variables and reactor design impact the effectiveness of pathogen kills. The microbial killing mechanisms of ED, including the role of free radicals and the electric field (EF), are inherently harmful to microorganisms. Additionally, other mechanisms have been proposed to explain the timeframes for implementing ED in different applications. The processes involved in ED can be categorized into four pathways. The first pathway involves oxidative stress and cell death caused by the production of oxidants. The second pathway is the cell membranes' irreparable permeabilization due to the used EF. The third pathway is the electrooxidation of critical cellular components when exposed to electric current or induced EFs. Lastly, the fourth pathway is the electrosorption of negatively charged bacteria onto the anode, pursued by a direct electron transfer reaction. The coming exploration must concentrate on comprehending the impact of EF on ED and implementing safe multi-hurdle methods such as distillation, plasma discharge, nanotechnologies, and membrane processes in industrial settings. Granular activated carbon is recommended as a post-treatment method to reduce the concentrations of disinfection by-products (DBPs). Adsorptive techniques and membrane processes remain promising research areas due to their comparatively small prices and simplicity of application. Further investigations into improving electrochemical reactors and optimizing electrolysis conditions are necessary. Integrating the ED process with different treatment methods guarantees improved disinfection effectiveness, reduced power use, and minimized DBPs. This review encourages combining more than two processes simultaneously or consecutively for better efficiency.
References
Ramírez-Castillo FY, Loera-Muro A, Jacques M, Garneau P, Avelar-González FJ, Harel J, et al. Waterborne pathogens: detection methods and challenges. Pathogens. 2015;4:307-334. https://www.mdpi.com/2076-0817/4/2/307.
Li Z, Yang D, Li S, Yang L, Yan W, Xu H. Advances on electrochemical disinfection research: Mechanisms, influencing factors and applications. Sci Total Environ. 2024;912:169043. https://doi.org/10.1016/j.scitotenv.2023.169043.
Albolafio S, Marín A, Allende A, García F, Simón-Andreu PJ, Soler MA, et al. Strategies for mitigating chlorinated disinfection byproducts in wastewater treatment plants. Chemosphere. 2022;288:132583. https://doi.org/10.1016/j.chemosphere.2021.132583.
Soltermann F, Abegglen C, Tschui M, Stahel S, von Gunten U. Options and limitations for bromate control during ozonation of wastewater. Water Res. 2017;116:76-85. https://doi.org/10.1016/j.watres.2017.02.026.
Lu X, Zhou X, Qiu W, Wang Z, Cheng H, Zhang H, et al. Singlet oxygen involved electrochemical disinfection by anodic oxidation of H2O2 in the presence of Cl−. Chem Eng J. 2022;446:136871. https://doi.org/10.1016/j.cej.2022.136871.
Antonelli M, Mezzanotte V, Nurizzo C. Wastewater disinfection by UV irradiation: Short and long-term efficiency. Environ Eng Sci. 2008;25:363-373. https://doi.org/10.1089/ees.2006.0268.
Bharti B, Li H, Ren Z, Zhu R, Zhu Z. Recent advances in sterilization and disinfection technology: A review. Chemosphere. 2022;308:136404. https://doi.org/10.1016/j.chemosphere.2022.136404.
Xu J, Yang L, Zhou X. A systematical review of blackwater treatment and resource recovery: Advance in technologies and applications. Resour Conserv Recycl. 2023;197:107066. https://doi.org/10.1016/j.resconrec.2023.107066.
Ghasemian S, Asadishad B, Omanovic S, Tufenkji N. Electrochemical disinfection of bacteria-laden water using antimony-doped tin-tungsten-oxide electrodes. Water Res. 2017;126:299-307. https://doi.org/10.1016/j.watres.2017.09.029.
Gonzalez-Rivas N, Reyes-Pérez H, Barrera-Díaz CE. Recent advances in water and wastewater electrodisinfection. Chem Electro Chem. 2019;6:1978. https://doi.org/10.1002/celc.201801746.
Hand S, Cusick RD. Electrochemical disinfection in water and wastewater treatment: Identifying impacts of water quality and operating conditions on performance. Environ Sci Technol. 2021;55:3470-3482. https://doi.org/10.1021/acs.est.0c06254.
Bergmann H. Electrochemical disinfection – State of the art and tendencies. Curr Opin Electrochem. 2021;28:100694. https://doi.org/10.1016/j.coelec.2021.100694.
Wei S, Chen T, Hou H, Xu Y. Recent advances in electrochemical sterilization. J Electroanal Chem. 2023;937:117419. https://doi.org/10.1016/j.jelechem.2023.117419.
Feng C, Suzuki K, Zhao S, Sugiura N, Shimada S, Maekawa T. Water disinfection by electrochemical treatment. Bioresour Technol. 2004;94:21-25. https://doi.org/10.1016/j.biortech.2003.11.021.
Ghernaout D, Elboughdiri N, Ghernaout B, Ashraf GA, Benaissa M. Virus removal by iron coagulation processes. Green Sustain Chem. 2023;13:171-208. https://doi.org/10.4236/gsc.2023.133010.
Li Z-H, You S-J, Gong X-B, Wan X-Y, Ren N-Q. Effective electrochemical sterilization based on electrocatalysis of oxygen reduction by multiwalled carbon nanotubes. Chem Electro Chem. 2016;3:1678. https://doi.org/10.1002/celc.201600210.
Vecitis CD, Schnoor MH, Rahaman MS, Schiffman JD, Elimelech M. Electrochemical multiwalled carbon nanotube filter for viral and bacterial removal and inactivation. Environ Sci Technol. 2011;45:3672-3679. https://doi.org/10.1021/es2000062.
Guo L, Ding K, Rockne K, Duran M, Chaplin BP. Bacteria inactivation at a sub-stoichiometric titanium dioxide reactive electrochemical membrane. J Hazard Mater. 2016;319:137-146. https://doi.org/10.1016/j.jhazmat.2016.05.051.
Xie L, Shu Y, Hu Y, Cheng J, Chen Y. SWNTs-PAN/TPU/PANI composite electrospun nanofiber membrane for point-of-use efficient electrochemical disinfection: New strategy of CNT disinfection. Chemosphere. 2020;251:126286. https://doi.org/10.1016/j.chemosphere.2020.126286.
Garcia-Segura S, Keller J, Brillas E, Radjenovic J. Removal of organic contaminants from secondary effluent by anodic oxidation with a boron-doped diamond anode as tertiary treatment. J Hazard Mater. 2015;283:551-557. https://doi.org/10.1016/j.jhazmat.2014.10.003.
Moreno-Palacios AV, Palma-Goyes RE, Vazquez-Arenas J, Torres-Palma RA. Bench-scale reactor for Cefadroxil oxidation and elimination of its antibiotic activity using electro-generated active chlorine. J Environ Chem Eng. 2019;7:103173. https://doi.org/10.1016/j.jece.2019.103173.
de Moura DC, de Araújo CKC, Zanta CLPS, Salazar R, Martínez-Huitle CA. Active chlorine species electrogenerated on Ti/Ru0.3Ti0.7O2 surface: Electrochemical behavior, concentration determination and their application. J Electroanal Chem. 2014;731:145-152. https://doi.org/10.1016/j.jelechem.2014.08.008.
Wei S, Shi L, Ren Z, Zhang A, Ming R, Chai B, et al. Preparation of electrolyzed oxidizing water with a platinum electrode prepared by magnetron sputtering technique. RSC Adv. 2017;7:45377-84. https://doi.org/10.1039/C7RA08150B.
Kwon N, Chen Y, Chen X, Kim MH, Yoon J. Recent progress on small molecule-based fluorescent imaging probes for hypochlorous acid (HOCl)/hypochlorite (OCl−). Dyes Pigments. 2022;200:110132. https://doi.org/10.1016/j.dyepig.2022.110132.
Garcia-Segura S, Ocon JD, Chong MN. Electrochemical oxidation remediation of real wastewater effluents — A review. Process Saf Environ Prot. 2018;113:48-67. https://doi.org/10.1016/j.psep.2017.09.014.
Jin Y, Shi Y, Chen R, Chen X, Zheng X, Liu Y. Electrochemical disinfection using a modified reticulated vitreous carbon cathode for drinking water treatment. Chemosphere. 2019;215:380-7. https://doi.org/10.1016/j.chemosphere.2018.10.057.
Bruguera-Casamada C, Sirés I, Brillas E, Araujo RM. Effect of electrogenerated hydroxyl radicals, active chlorine and organic matter on the electrochemical inactivation of Pseudomonas aeruginosa using BDD and dimensionally stable anodes. Sep Purif Technol. 2017;178:224-31. https://doi.org/10.1016/j.seppur.2017.01.042.
Zhang Y, Zuo S, Zhang Y, Li M, Cai J, Zhou M. Disinfection of simulated ballast water by a flow-through electro-peroxone process. Chem Eng J. 2018;348:485-93. https://doi.org/10.1016/j.cej.2018.04.123.
Jeong J, Kim JY, Yoon J. The role of reactive oxygen species in the electrochemical inactivation of microorganisms. Environ Sci Technol. 2006;40:6117-22. https://doi.org/10.1021/es0604313.
Zhang J, Su P, Zhu X, Mao R, Yuan L, Li A, et al. Enhanced purification and disinfection of restaurant wastewater by electro-coagulation coupled with an electro-oxidation process: From lab scale to field scale. ACS ES&T Eng. 2023;3:932-43. https://doi.org/10.1021/acsestengg.2c00381.
Feng Y, Yang L, Liu J, Logan BE. Electrochemical technologies for wastewater treatment and resource reclamation. Environ Sci Water Res Technol. 2016;2:800-31. https://doi.org/10.1039/c5ew00289c.
Li Z, Meunier JD, Delvaux B. Goethite affects phytolith dissolution through clay particle aggregation and pH regulation. Geochim Cosmochim Acta. 2023;349:11-22. https://doi.org/10.1016/j.gca.2023.03.021.
Norra GF, Baptista-Pires L, Lumbaque EC, Borrego CM, Radjenovic J. Chlorine-free electrochemical disinfection using graphene sponge electrodes. Chem Eng J. 2022;430:132772. https://doi.org/10.1016/j.cej.2021.132772.
Czerwińska-Główka D, Krukiewicz K. A journey in the complex interactions between electrochemistry and bacteriology: From electroactivity to electromodulation of bacterial biofilms. Bioelectrochem. 2020;131:07401. https://doi.org/10.1016/j.bioelechem.2019.107401.
Napotnik TB, Polajžer T, Miklavčič D. Cell death due to electroporation – A review. Bioelectrochem. 2021;141:107871. https://doi.org/10.1016/j.bioelechem.2021.107871.
Kotnik T, Frey W, Sack M, Meglič SH, Peterka M, Miklavčič D. Electroporation-based applications in biotechnology. Trends Biotechnol. 2015;33:480-88. https://doi.org/10.1016/j.tibtech.2015.06.002.
Pi SY, Sun MY, Zhao YF, Chong YX, Chen D, Liu H. Electroporation-coupled electrochemical oxidation for rapid and efficient water disinfection with Co3O4 nanowire arrays-modified graphite felt electrodes. Chem Eng J. 2022;435:134967. https://doi.org/10.1016/j.cej.2022.134967.
Garcia PA, Ge Z, Moran JL, Buie CR. Microfluidic screening of electric fields for electroporation. Sci Rep. 2016;6:21238. https://doi.org/10.1038/srep21238.
Tian J, Feng H, Yan L, Yu M, Ouyang H, Li H, et al. A self-powered sterilization system with both instant and sustainable anti-bacterial ability. Nano Energy. 2017;36:241-49. https://doi.org/10.1016/j.nanoen.2017.04.030.
Ghernaout D, Elboughdiri N, Lajimi R. Electrocoagulation of Escherichia coli culture: Effects of temperature and cell concentration. Open Access Lib J. 2022;9:e8763. https://doi.org/10.4236/oalib.1108763.
Ghernaout D. Electric field (EF) in the core of the electrochemical (EC) disinfection. Open Access Lib J. 2020;7:e6587. https://doi.org/10.4236/oalib.1106587.
Ghernaout D. Electrocoagulation as a pioneering separation technology—Electric field role. Open Access Lib J. 2020;7:e6702. https://doi.org/10.4236/oalib.1106702.
Shang K, Qiao Z, Sun B, Fan X, Ai S. An efficient electrochemical disinfection of E. coli and S. aureus in drinking water using ferrocene–PAMAM–multiwalled carbon nanotubes–chitosan nanocomposite modified pyrolytic graphite electrode. J Solid State Electrochem. 2013;17:1685-91. https://doi.org/10.1007/s10008-013-2031-5.
Li Z, Li X, Li S, Yang Y, Yan W, Xu H. Bibliometric analysis of electrochemical disinfection: Current status and development trend from 2002 to 2022. Environ Sci Pollut Res. 2023;30:111714-31. https://doi.org/10.1007/s11356-023-30117-3.
Matsunaga T, Nakasono S, Masuda S. Electrochemical sterilization of bacteria adsorbed on granular activated carbon. FEMS Microbiol Lett. 1992;93:255-60. https://doi.org/10.1016/0378-1097(92)90471-Y.
Ni XY, Liu H, Xin L, Xu ZB, Wang YH, Peng L, et al. Disinfection performance and mechanism of the carbon fiber-based flow-through electrode system (FES) towards Gram-negative and Gram-positive bacteria. Electrochim Acta. 2020;341:135993. https://doi.org/10.1016/j.electacta.2020.135993.
Murugesan P, Moses JA, Anandharamakrishnan C. Performance of an atmospheric plasma discharge reactor for inactivation of Enterocococcus faecalis and Escherichia coli in aqueous media. J Environ Chem Eng. 2020;8:103891. https://doi.org/10.1016/j.jece.2020.103891.
Zhang Z, Huang Z, Tong J, Wu Q, Pan Y, Malakar PK, Zhao Y. An outlook for food sterilization technology: targeting the outer membrane of foodborne gram-negative pathogenic bacteria. Curr Opin Food Sci. 2021;42:15-22. https://doi.org/10.1016/j.cofs.2021.02.013.
2. Ghernaout D, Elboughdiri N, Ghareba S, Salih A. Disinfecting water with the carbon fiber-based flow-through electrode system (FES): Towards axial dispersion and velocity profile. Open Access Lib J. 2020;7:e6238. https://doi.org/10.4236/oalib.1106238.
Ghernaout D, Elboughdiri N. Antibiotics resistance in water mediums: Background, facts, and trends. Appl Eng. 2020;4:1-6. https://doi.org/10.11648/j.ae.20200401.11.
Ghernaout D. Demobilizing antibiotic-resistant bacteria and antibiotic resistance genes by electrochemical technology: New insights. Open Access Lib J. 2020;7:e6685. https://doi.org/10.4236/oalib.1106685.
Ghernaout D, Elboughdiri N. Removing antibiotic-resistant bacteria (ARB) carrying genes (ARGs): Challenges and future trends. Open Access Lib J. 2020;7:e6003. https://doi.org/10.4236/oalib.1106003.
Huang JJ, Hu HY, Wu YH, Wei B, Lu Y. Effect of chlorination and ultraviolet disinfection on tetA-mediated tetracycline resistance of Escherichia coli. Chemosphere. 2013;90:2247-53. https://doi.org/10.1016/j.chemosphere.2012.10.008.
Allard JD, Bertrand KP. Membrane topology of the pBR322 tetracycline resistance protein. TetA-PhoA gene fusions and implications for the mechanism of TetA membrane insertion. J Biol Chem. 1992;267:17809-19. https://doi.org/10.1016/S0021-9258(19)37116-9.
Poole K. Stress responses as determinants of antimicrobial resistance in Pseudomonas aeruginosa: multidrug efflux and more. Can J Microbiol. 2014;60:783-91. https://doi.org/10.1139/cjm-2014-0666.
Michaelis C, Grohmann E. Horizontal gene transfer of antibiotic resistance genes in biofilms. Antibiotics. 2023;12:328. https://doi.org/10.3390/antibiotics12020328.
Zhang C, Zhao X, Wang C, Hakizimana I, Crittenden JC, Laghari AA. Electrochemical flow-through disinfection reduces antibiotic resistance genes and horizontal transfer risk across bacterial species. Water Res. 2022;212:118090. https://doi.org/10.1016/j.watres.2022.118090.
Liu Y, Zhang S, Fang H, Wang Q, Jiang S, Zhang C, Qiu P. Inactivation of antibiotic resistant bacterium Escherichia coli by electrochemical disinfection on molybdenum carbide electrode. Chemosphere. 2022;287:132398. https://doi.org/10.1016/j.chemosphere.2021.132398.
Zuo X, Cao WX, Li Y, Wang T. Antibiotic resistant bacteria inactivation through metal-free electrochemical disinfection with carbon catalysts and its potential risks. Chemosphere. 2022;305:135496. https://doi.org/10.1016/j.chemosphere.2022.135496.
Li H, Zhang Z, Duan J, Li N, Li B, Song T, Sardar MF, Lv X, Zhu C. Electrochemical disinfection of secondary effluent from a wastewater treatment plant: Removal efficiency of ARGs and variation of antibiotic resistance in surviving bacteria. Chem Eng J. 2020;392:123674. https://doi.org/10.1016/j.cej.2019.123674.
Meng LX, Sun YJ, Zhu L, Lin ZJ, Shuai XY, Zhou ZC, Chen H. Mechanism and potential risk of antibiotic resistant bacteria carrying last resort antibiotic resistance genes under electrochemical treatment. Sci Total Environ. 2022;821:153367. https://doi.org/10.1016/j.scitotenv.2022.153367.
Mousazadeh M, Kabdaşlı I, Khademi S, Sandoval MA, Moussavi SP, Malekdar F, Gilhotra V, Hashemi M, Dehghani MH. A critical review on the existing wastewater treatment methods in the COVID-19 era: What is the potential of advanced oxidation processes in combatting viral especially SARS-CoV-2?. J Water Process Eng. 2022;49:103077. https://doi.org/10.1016/j.jwpe.2022.103077.
Ghernaout D, Elboughdiri N. Searching if SARS-CoV-2 subsists following the disinfection of potable water. Open Access Lib J. 2021;8:e7505. https://doi.org/10.4236/oalib.1107505.
Tu Y, Tang W, Yu L, Liu Z, Liu Y, Xia H, Zhang H, Chen S, Wu J, Cui X, Zhang J, Wang F, Hu Y, Deng D. Inactivating SARS-CoV-2 by electrochemical oxidation. Sci Bull. 2021;66:720-6. https://doi.org/10.1016/j.scib.2020.12.025.
Buonerba A, Corpuz MVA, Ballesteros F, Choo KH, Hasan SW, Korshin GV, Belgiorno V, Barceló D, Naddeo V. Coronavirus in water media: Analysis, fate, disinfection and epidemiological applications. J Hazard Mater. 2021;415:125580. https://doi.org/10.1016/j.jhazmat.2021.125580.
Drees KP, Abbaszadegan M, Maier RM. Comparative electrochemical inactivation of bacteria and bacteriophage. Water Res. 2003;37:2291-300. https://doi.org/10.1016/S0043-1354(03)00009-5.
Nair AM, Kumar A, Barbhuiya NH, Singh SP. Electrochemical inactivation of enteric viruses MS2, T4, and Phi6 using doped laser-induced graphene electrodes and filters. Environ Sci Nano. 2023;10:2077-89. https://doi.org/10.1039/D3EN00124E.
Yang C, Wen L, Li Y, Li XY. Fabrication of SnO2-Sb reactive membrane electrodes for high-efficiency electrochemical inactivation of bacteria and viruses in water. Chem Eng J. 2022;446:137327. https://doi.org/10.1016/j.cej.2022.137327.
Heffron J, Ryan DR, Mayer BK. Sequential electrocoagulation-electrooxidation for virus mitigation in drinking water. Water Res. 2019;160:435-44. https://doi.org/10.1016/j.watres.2019.05.078.
Ghernaout D, Elboughdiri N. Modeling viruses’ isoelectric points as a milestone in intensifying the electrocoagulation process for their elimination. Open Access Lib J. 2021;8:e7166. https://doi.org/10.4236/oalib.1107166.
Ghernaout D, Elboughdiri N. On the other side of viruses in the background of water disinfection. Open Access Lib J. 2020;7:e6374. https://doi.org/10.4236/oalib.1106374.
Wigginton KR, Pecson BM, Sigstam T, Bosshard F, Kohn T. Virus inactivation mechanisms: Impact of disinfectants on virus function and structural integrity. Environ Sci Technol. 2012;46:12069-78. https://doi.org/10.1021/es3029473.
Fitch A, Balderas-Hernandez P, Ibanez JG. Electrochemical technologies combined with physical, biological, and chemical processes for the treatment of pollutants and wastes: A review. J Environ Chem Eng. 2022;10:107810. https://doi.org/10.1016/j.jece.2022.107810.
Zhou J, Hung YC, Xie X. Making waves: Pathogen inactivation by electric field treatment: From liquid food to drinking water. Water Res. 2021;207:117817. https://doi.org/10.1016/j.watres.2021.117817.
Pi SY, Wang Y, Lu YW, Liu GL, Wang DL, Wu HM, Chen D, Liu H. Fabrication of polypyrrole nanowire arrays-modified electrode for point-of-use water disinfection via low-voltage electroporation. Water Res. 2021;207:117825. https://doi.org/10.1016/j.watres.2021.117825.
Wang T, Brown DK, Xie X. Operando investigation of locally enhanced electric field treatment (LEEFT) harnessing lightning-rod effect for rapid bacteria inactivation. Nano Lett. 2022;22:860-7. https://doi.org/10.1021/acs.nanolett.1c02240.
Zhou J, Wang T, Xie X. Locally enhanced electric field treatment (LEEFT) promotes the performance of ozonation for bacteria inactivation by disrupting the cell membrane. Environ Sci Technol. 2020;54:14017-25. https://doi.org/10.1021/acs.est.0c03968.
Lu YW, Liang XX, Wang CY, Chen D, Liu H. Synergistic nanowire-assisted electroporation and chlorination for inactivation of chlorine-resistant bacteria in drinking water systems via inducing cell pores for chlorine permeation. Water Res. 2023;229:119399. https://doi.org/10.1016/j.watres.2022.119399.
Huo ZY, Winter LR, Wang XX, Du Y, Wu YH, Hübner U, Hu HY, Elimelech M. Synergistic nanowire-enhanced electroporation and electrochlorination for highly efficient water disinfection. Environ Sci Technol. 2022;56:10925-34. https://doi.org/10.1021/acs.est.2c01793.
Liu H, Huang W, Yu Y, Chen D. Lightning-rod effect on nanowire tips reinforces electroporation and electrochemical oxidation: An efficient strategy for eliminating intracellular antibiotic resistance genes. ACS Nano. 2023;17:3037-46. https://doi.org/10.1021/acsnano.2c11811.
da Costa PRF, de A Costa ECT, Castro SSL, Fajardo AS, Martínez-Huitle CA. A sequential process to treat a cashew-nut effluent: Electrocoagulation plus electrochemical oxidation. J Electroanal Chem. 2019;834:79-85. https://doi.org/10.1016/j.jelechem.2018.12.035.
Ghernaout D, Badis A, Ghernaout B, Kellil A. Application of electrocoagulation in Escherichia coli culture and two surface waters. Desalination. 2008;219:118-25. https://doi.org/10.1016/j.desal.2007.05.010.
Ghernaout D, Elboughdiri N. Electrocoagulation as a pretreatment of electrooxidation for killing Escherichia coli. Open Access Lib J. 2024;11:e11271. https://doi.org/10.4236/oalib.1111271.
Fan T, Deng W, Feng X, Pan F, Li Y. An integrated electrocoagulation – Electrocatalysis water treatment process using stainless steel cathodes coated with ultrathin TiO2 nanofilms. Chemosphere. 2020;254:126776. https://doi.org/10.1016/j.chemosphere.2020.126776.
Lynn W, Heffron J, Mayer BK. Electrocoagulation as a pretreatment for electrooxidation of E. coli. Water. 2019;11:2509. https://doi.org/10.3390/w11122509.
Cotillas S, Llanos J, Cañizares P, Mateo S, Rodrigo MA. Optimization of an integrated electrodisinfection/electrocoagulation process with Al bipolar electrodes for urban wastewater reclamation. Water Res. 2013;47:1741-50. https://doi.org/10.1016/j.watres.2012.12.029.
Llanos J, Cotillas S, Cañizares P, Rodrigo MA. Effect of bipolar electrode material on the reclamation of urban wastewater by an integrated electrodisinfection/electrocoagulation process. Water Res. 2014;53:329-38. https://doi.org/10.1016/j.watres.2014.01.041.
Cotillas S, Llanos J, Moraleda I, Cañizares P, Rodrigo MA. Scaling-up an integrated electrodisinfection-electrocoagulation process for wastewater reclamation. Chem Eng J. 2020;380:122415. https://doi.org/10.1016/j.cej.2019.122415.
Alighardashi A, Aghta RS, Ebrahimzadeh H. Improvement of carbamazepine degradation by a three-dimensional electrochemical (3-EC) process. Int J Environ Res. 2018;12:451-8. https://doi.org/10.1007/s41742-018-0102-2.
Rogers TW, Rogers TS, Stoner MH, Sellgren KL, Lynch BJ, Forbis-Stokes AA, Stoner BR, Hawkins BT. A granular activated carbon/electrochemical hybrid system for onsite treatment and reuse of blackwater. Water Res. 2018;144:553-60. https://doi.org/10.1016/j.watres.2018.07.070.
Mesones S, Mena E, López-Muñoz MJ, Adán C, Marugán J. Synergistic and antagonistic effects in the photoelectrocatalytic disinfection of water with TiO2 supported on activated carbon as a bipolar electrode in a novel 3D photoelectrochemical reactor. Sep Purif Technol. 2020;247:117002. https://doi.org/10.1016/j.seppur.2020.117002.
Ma J, Gao M, Shi H, Ni J, Xu Y, Wang Q. Progress in research and development of particle electrodes for three-dimensional electrochemical treatment of wastewater: a review. Environ Sci Pollut Res. 2021;28:47800-24. https://doi.org/10.1007/s11356-021-13785-x.
Liu H, Wu YH, Ni XY, Chen Z, Peng L, Huo ZY, Wang Q, Hu HY. Construction and optimization mechanisms of carbon fiber-based flow-through electrode system (FES) with stackable multi-cathode units for water disinfection. J Hazard Mater. 2020;399:123065. https://doi.org/10.1016/j.jhazmat.2020.123065.
Hussain SN, Trzcinski AP, Asghar HMA, Sattar H, Brown NW, Roberts EPL. Disinfection performance of adsorption using graphite adsorbent coupled with electrochemical regeneration for various microorganisms present in water. J Ind Eng Chem. 2016;44:216-25. https://doi.org/10.1016/j.jiec.2016.09.009.
Andrés García E, Agulló-Barceló M, Bond P, Keller J, Gernjak W, Radjenovic J. Hybrid electrochemical-granular activated carbon system for the treatment of greywater. Chem Eng J. 2018;352:405-11. https://doi.org/10.1016/j.cej.2018.07.042.
Hussain SN, de las Heras N, Asghar HMA, Brown NW, Roberts EPL. Disinfection of water by adsorption combined with electrochemical treatment. Water Res. 2014;54:170-8. https://doi.org/10.1016/j.watres.2014.01.043.
Li Y, Kemper JM, Datuin G, Akey A, Mitch WA, Luthy RG. Reductive dehalogenation of disinfection byproducts by an activated carbon-based electrode system. Water Res. 2016;98:354-62. https://doi.org/10.1016/j.watres.2016.04.019.
Jiang J, Zhang X. A smart strategy for controlling disinfection byproducts by reversing the sequence of activated carbon adsorption and chlorine disinfection. Sci Bull. 2018;63:1167-1169. https://doi.org/10.1016/j.scib.2018.07.022.
Gil MI, López-Gálvez F, Andújar S, Moreno M, Allende A. Disinfection by-products generated by sodium hypochlorite and electrochemical disinfection in different process wash water and fresh-cut products and their reduction by activated carbon. Food Control. 2019;100:46-52. https://doi.org/10.1016/j.foodcont.2018.12.050.
Hassani A, Malhotra M, Karim AV, Krishnan S, Nidheesh PV. Recent progress on ultrasound-assisted electrochemical processes: A review on mechanism, reactor strategies, and applications for wastewater treatment. Environ Res. 2022;205:112463. https://doi.org/10.1016/j.envres.2021.112463.
Lazarotto JS, Júnior EPM, Medeiros RC, Volpatto F, Silvestri S. Sanitary sewage disinfection with ultraviolet radiation and ultrasound. Int J Environ Sci Technol. 2022;19:11531-11538. https://doi.org/10.1007/s13762-021-03764-7.
Ritesh P, Srivastava VC. Understanding of ultrasound enhanced electrochemical oxidation of persistent organic pollutants. J Water Proc Eng. 2020;37:101378. https://doi.org/10.1016/j.jwpe.2020.101378.
Blume T, Neis U. Improving chlorine disinfection of wastewater by ultrasound application. Water Sci Technol. 2005;52:139-144. https://doi.org/10.2166/wst.2005.0687.
Joyce E, Mason TJ, Phull SS, Lorimer JP. The development and evaluation of electrolysis in conjunction with power ultrasound for the disinfection of bacterial suspensions. Ultrason Sonochem. 2003;10:231-234. https://doi.org/10.1016/S1350-4177(03)00109-3.
Ghernaout D, Elboughdiri N. Electrocoagulation process in the context of disinfection mechanism. Open Access Lib J. 2020;7:e6083. https://doi.org/10.4236/oalib.1106083.
Llanos J, Cotillas S, Cañizares P, Rodrigo MA. Conductive diamond sono-electrochemical disinfection (CDSED) for municipal wastewater reclamation. Ultrason Sonochem. 2015;22:493-498. https://doi.org/10.1016/j.ultsonch.2014.05.009.
Gibson JH, Yong DHN, Farnood RR, Seto P. A literature review of ultrasound technology and its application in wastewater disinfection. Water Qual Res J. 2008;43:23-35. https://doi.org/10.2166/wqrj.2008.004.
Cotillas S, Llanos J, Castro-Ríos K, Taborda-Ocampo G, Rodrigo MA, Cañizares P. Synergistic integration of sonochemical and electrochemical disinfection with DSA anodes. Chemosphere. 2016;163:562-568. https://doi.org/10.1016/j.chemosphere.2016.08.034.
Ninomiya K, Arakawa M, Ogino C, Shimizu N. Inactivation of Escherichia coli by sonoelectrocatalytic disinfection using TiO2 as electrode. Ultrason Sonochem. 2013;20:762-767. https://doi.org/10.1016/j.ultsonch.2012.10.007.
Patidar R, Srivastava VC. Ultrasound-assisted enhanced electroxidation for mineralization of persistent organic pollutants: A review of electrodes, reactor configurations and kinetics. Crit Rev Environ Sci Technol. 2021;51:1667-1701. https://doi.org/10.1080/10643389.2020.1769427.
Ingelsson M, Yasri N, Roberts EP. Electrode passivation, faradaic efficiency, and performance enhancement strategies in electrocoagulation—a review. Water Research. 2020;187:116433. https://doi.org/10.1016/j.watres.2020.116433.
Saravanan A, Senthil Kumar P, Jeevanantham S, Karishma S, Kiruthika AR. Photocatalytic disinfection of micro-organisms: Mechanisms and applications. Environ Technol Innov. 2021;24:101909. https://doi.org/10.1016/j.eti.2021.101909.
113. Shi F, Ding M, Tong H, Yang Y, Zhang J, Wang L, Li H, Huo Y. Photoelectrocatalytic sterilization on thorn-like ZIF-67/ZnO hybrid photoanodes. J Environ Chem Eng. 2022;10:107385. https://doi.org/10.1016/j.jece.2022.107385.
Liu Y, Gan X, Zhou B, Xiong B, Li J, Dong C, Bai J, Cai W. Photoelectrocatalytic degradation of tetracycline by highly effective TiO2 nanopore arrays electrode. J Hazard Mater. 2009;171:678-683. https://doi.org/10.1016/j.jhazmat.2009.06.054.
Ghernaout D, Elboughdiri N, Lajimi R. E. coli: Health impacts, exposure evaluation, and hazard reduction. Open Access Lib J. 2022;9:e8860. https://doi.org/10.4236/oalib.1108860.
Wang N, Li X, Wang Y, Quan X, Chen G. Evaluation of bias potential enhanced photocatalytic degradation of 4-chlorophenol with TiO2 nanotube fabricated by anodic oxidation method. Chem Eng J. 2009;146:30-35. https://doi.org/10.1016/j.cej.2008.05.025.
Daghrir R, Drogui P, Robert D. Photoelectrocatalytic technologies for environmental applications. J Photochem Photobiol A Chem. 2012;238:41-52. https://doi.org/10.1016/j.jphotochem.2012.04.009.
Sun H, Li G, Nie X, Shi H, Wong P-K, Zhao H, An T. Systematic approach to in-depth understanding of photoelectrocatalytic bacterial inactivation mechanisms by tracking the decomposed building blocks. Environ Sci Technol. 2014;48:9412-9419. https://doi.org/10.1021/es502471h.
Philippidis N, Nikolakaki E, Sotiropoulos S, Poulios I. Photoelectrocatalytic inactivation of E. coli XL 1 blue colonies in water. J Chem Technol Biotechnol. 2010;85:1054-1060. https://doi.org/10.1002/jctb.2394.
Domínguez-Espíndola RB, Bruguera-Casamada C, Silva-Martínez S, Araujo RM, Brillas E, Sirés I. Photoelectrocatalytic inactivation of Pseudomonas aeruginosa using an Ag-decorated TiO2 photoanode. Sep Purif Technol. 2019;208:83-91. https://doi.org/10.1016/j.seppur.2018.05.005.
Garcia-Segura S, Brillas E. Advances in solar photoelectro-Fenton: Decolorization and mineralization of the Direct Yellow 4 diazo dye using an autonomous solar pre-pilot plant. Electrochim Acta. 2014;140:384-395. https://doi.org/10.1016/j.electacta.2014.04.009.
Ranga M, Sinha S. Mechanism and techno-economic analysis of the electrochemical process. Chem Rev. 2023;10:336-362. https://doi.org/10.1002/cben.202200025.
Sun M, Wang X, Winter LR, Zhao Y, Ma W, Hedtke T, Kim J-H, Elimelech M. Electrified membranes for water treatment applications. ACS ES&T Eng. 2021;1:725-752. https://doi.org/10.1021/acsestengg.1c00015.
Ghernaout D, Elboughdiri N, Lajimi R. Combining electrified membranes and electrochemical disinfection for virus demobilization. Open Access Lib J. 2022;9:e8749. https://doi.org/10.4236/oalib.1108749.
McHugh PJ, Stergiou AD, Symes MD. Decoupled electrochemical water splitting: From fundamentals to applications. Adv Energy Mater. 2020;10:2002453. https://doi.org/10.1002/aenm.202002453.
Ghernaout D, Elboughdiri N. Towards combining electrochemical water splitting and electrochemical disinfection. Open Access Lib J. 2021;8:e7445. https://doi.org/10.4236/oalib.1107445.
Wallace AG, Symes MD. Decoupling strategies in electrochemical water splitting and beyond. Joule. 2018;2:1390-1395. https://doi.org/10.1016/j.joule.2018.06.011.
Ghernaout D, Elboughdiri N, Ghareba S, Salih A. Electrochemical advanced oxidation processes (EAOPs) for disinfecting water – Fresh perspectives. Open Access Lib J. 2020;7:e6257. https://doi.org/10.4236/oalib.1106257.
Ganiyu SO, Martínez-Huitle CA, Rodrigo MA. Renewable energies driven electrochemical wastewater/soil decontamination technologies: A critical review of fundamental concepts and applications. Appl Catal B Environ. 2020;270:118857. https://doi.org/10.1016/j.apcatb.2020.118857.
Ghernaout D. Water treatment challenges towards viruses removal. Open Access Lib J. 2020;7:e6408. https://doi.org/10.4236/oalib.1106408.
Gerba CP, Pepper IL. Drinking water treatment and distribution, Ch. 28. In: Pepper IL, Gerba CP, Gentry TJ, eds. Environmental Microbiology. 3rd ed. Elsevier Inc.; 2015:633-643. https://doi.org/10.1016/B978-0-12-394626-3.00028-4.
Martínez-Huitle CA, Brillas E. A critical review over the electrochemical disinfection of bacteria in synthetic and real wastewaters using a boron-doped diamond anode. Curr Opin Sol State Mater Sci. 2021;25:100926. https://doi.org/10.1016/j.cossms.2021.100926.
Ghernaout D, Elboughdiri N. Disinfecting water: Plasma discharge for removing coronaviruses. Open Access Lib J. 2020;7:e6314. https://doi.org/10.4236/oalib.1106314.
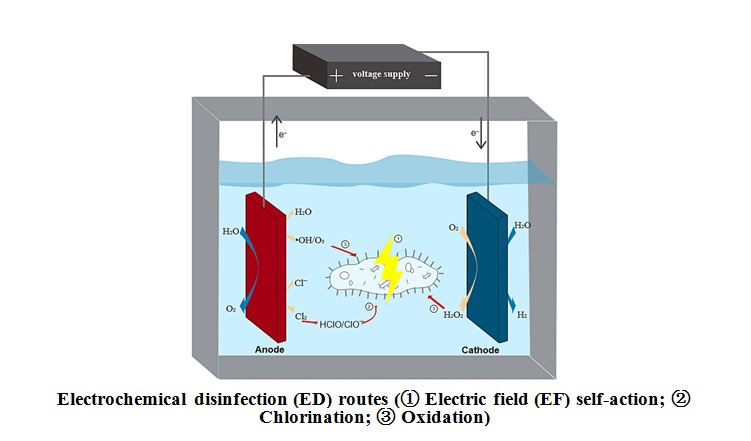
Downloads
Published
How to Cite
Issue
Section
License
Copyright (c) 2024 Djamel Ghernaout , Noureddine Elboughdiri , Badia Ghernaout, Mhamed Benaissa, Alsamani Salih

This work is licensed under a Creative Commons Attribution-NonCommercial 4.0 International License.